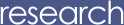
Mechanisms underlying plant adaptation to salt stress
One of the most urgent global problems is finding enough water and land to support the world’s food needs. The increasing demand for plant-based agricultural commodities will require improved productivity from land currently in cultivation as well as expansion into marginal land for agricultural use (6). In either case, one of the biggest problems facing agriculture is increased salinity in the soil solution. Salinity affects land that receives little rain (due to insufficient water to leach salts) and is a growing problem for irrigation agriculture (because long-term irrigation leads to the accumulation of salts; 3, 7, 8). Improved cultural practices alone will not be enough to keep up with the growing demand for food -- identification of plant stress tolerance elements and the underlying adaptive mechanisms will be needed to produce crops that maintain yield in increasingly saline environments.
Recently, Thellungiella halophila (synonymous with Thellungiella salsuginea, salt cress), a salt-tolerant relative of both Arabidopsis and the agriculturally important Brassica, was identified in the saline environments of coastal China and the Canadian prairies (1). T. halophila is becoming a key model plant for understanding adaptation to salt stress based on several characteristics. First, unlike many halophytes, T. halophila does not require salt for optimal growth but is able to survive in levels of salt as high as 500 mM NaCl (soil treatment) without relying on spec ialized morphological features (Fig. 1 – soil grown At vs.Th). Instead, salt tolerance in T. halophila appears to be a function of regulatory changes in basic biochemical andphysiological mechanisms also present in salt-sensitive plants. Transfer of such adaptations to crop plants is anticipated to be far easier and more successful than transfer of complicated morphological adaptations. Second, T. halophila is a diploid plant amenable to genetic studies, primarily due to its ability to be transformed via Agrobacterium at rates comparable to those achieved in Arabidopsis (2). Finally, T. halophila shares numerous key characteristics with other model plants that facilitate rapid and efficient genetic analysis, including a small genome (estimated to be approximately twice that of Arabidopsis), small stature, self-pollination, short life cycle and prolific seed production.
With funding from the Southwest Consortium on Plant Genetics and Water Resources and in collaboration with Dr. Richard Jorgensen (Department of Plant Sciences, University of Arizona), we are taking a forward genetic approach to identify genes responsible for T. halophila’s salt tolerance. T. halophila mutants with altered salt sensitivity are being generated by sense RNA interference (S-RNAi), a gene silencing technique (4, 5). S-RNAi induces silencing by overexpression of a sense RNA homologous to the target transcript. This sense RNA is converted to double-stranded RNA (dsRNA) by the action of an endogenous RNA-directed RNA polymerase. The dsRNA then triggers gene silencing of the target transcript. The major advantage of this type of RNA interference is that we can clone a select population of complementary DNA (cDNA) sequences into an appropriate vector to produce a pool of S-RNAi constructs targeting only genes in this specific group. Transformation of plants with this population of S-RNAi constructs leads to a cohort of transgenic plants in which each individual is likely to be mutagenized by silencing of a target gene represented in the cDNA population. For our studies, a set of T. halophila salt-induced genes are the preferential targets; this should dramatically increase the efficiency of genetic analysis of salt tolerance in this organism. These studies will enable us to functionally identify the genes, the biochemical mechanisms and the signaling pathways that mediate tolerance in a halophyte.
In collaboration with Dr. Rod Wing (Department of Plant Sciences, University of Arizona) and Dr. Thomas Mitchell-Olds (Department of Biology, Duke University) we are working with the Department of Energy-Joint Genome Institute to sequence the T. halophila genome. Having access to its genome sequence in combination with functional genetic approaches will provide unprecedented insight into the molecular signaling networks that underlie T. halophila’s tolerance to high levels of soil salinity. In addition to facilitating our understanding of tolerance at the cellular and whole plant level, comparative studies of the genomes of Arabidopsis and T. halophila will allow us to identify elements that have led to two closely related plant species with differing abiotic stress adaptation as well as to test the functions of these alternative alleles.
________________________________
REFERENCES
1. Amtmann A, Bohnert H, Bressan R. 2005. Abiotic stress and plant genome evolution. Search for new models. Plant Physiol 138: 127-30.
2. Bressan RA, Zhang C, Zhang H, Hasegawa PM, Bohnert HJ, Zhu J-K. 2001. Learning from the Arabidopsis experience. The next gene search paradigm. Plant Physiol 127: 1354-60.
3. Evans, L.T. 1998. Feeding the Ten Billion. Cambridge University Press.
4. Jorgensen RA. 2003. Sense cosuppression in plants: past, present and future. In: RNAi: a guide to gene silencing. Hannon GJ (ed). Cold Spring Harbor Laboratory Press, Cold Spring, New York. Pp. 5-21.
5. Napoli CA, Lemieux C, Jorgensen RA. 1990. Introduction of a chimeric chalcone synthase gene into petunia results in reversible co-suppression of homologous genes in trans. Plant Cell 2, 279-89.
6. O’Leary JW. 1995. Adaptive Components of salt tolerance. In: Handbook of plant and crop physiology. Mohammad Pessarakli, ed. Marcel Dekker, Inc, NY, pp. 577-85.
7. Penning de Vries FWT. 2001. Food security? We are loosing ground fast! In: Nösberger J et al., eds. Crop Science: Progress and prospects, CABI Internatl Publ., pp. 1-14.
8. Rhoades JD, Loveday J. 1990. Salinity in irrigated agriculture. In: American Society of Civil Engineers, Irrigation of Agricultural Crops (Steward, B.A. and Nielsen, D.R., eds), Am. Soc. Agronomists, Monograph 30, 1089-1142.
home | who we are | research | publications | tools | contact us
|