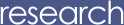
We are interested in understanding how plants control cellular levels of both beneficial and toxic ions during normal growth and development and when the plant experiences an abiotic stress. Our research activities are focused in the following two areas. Abiotic stress - Mechanisms underlying plant adaptation to salt stress . Environmental stresses such as salinity limit crop productivity worldwide. Sodium enters plant cells and inactivates metabolic enzymes leading to cell death and ultimately to death of the plant. Maintaining low levels of sodium in the cell is crucial for plant growth and development. Through comparative studies with various ecotypes of Arabidopsis thaliana (a salt-sensitive plant, a glycophyte) and a related species, Thellungiella halophila (a salt-tolerance plant, a halophyte), our goal is to elucidate the molecular basis of plant salt tolerance.
Signal transduction - Mechanisms controlling stimulus-induced changes in intracellular calcium .
Calcium (Ca2+) has emerged as a critical component of many pathways in plants, underlying growth and development by linking perception of physiological and environmental cues to cellular responses. Because cellular Ca2+ levels are tightly regulated, small changes in cytosolic Ca2+ levels provide information for the modification of enzyme activity and gene expression needed for the subsequent responses. Changes in intracellular Ca2+ are generated through the combined activities of passive transporters that allow Ca2+ to enter the cell and active transporters that utilize energy to remove Ca2+ from the cytoplasm. We are using Arabidopsis thaliana to identify passive calcium transporters that regulate early events in stimulus-induced changes in plant growth and development.
Mechanisms underlying plant adaptation to salt stress
One of the most urgent global problems is finding enough water and land to support the world's food needs. The increasing demand for plant-based agricultural commodities will require improved productivity from land currently in cultivation as well as expansion into marginal land for agricultural use (1). In either case, one of the biggest problems facing agriculture is increased salinity in the soil solution. Salinity affects land that receives little rain ( due to insufficient water to leach salts) and is a growing problem for irrigation agriculture (because long-term irrigation inevitably leads to the accumulation of salts; 2-4). [Figure 1 – salty field] Improved cultural practices alone will not be enough to keep up with the growing demand for food -- identification of plant stress tolerance elements and the underlying adaptive mechanisms will be needed to produce crops that maintain yield in increasingly saline environments. Using Arabidopsis thaliana (a plant that is sensitive to growth in salt, a glycophyte), we are combining genetic and biochemical approaches to identify these tolerance elements and adaptive mechanisms. Using Thellungiella halophila (a plant that is adapted to growth in salt, a halophyte), we are combining reverse genetic and biochemical approaches to determine if the salt tolerance determinants and mechanisms identified in Arabidopsis are part of the adaptations used by a naturally salt tolerant plant.
Molecular mechanisms underlying regulation of cellular Na+ ion homeostasis during plant growth in salt
Accumulation of Na+ in the cell cytoplasm of plants is toxic because of its adverse effects on cellular metabolism and ion homeostasis. In order to avoid the harmful effects of salt stress on growth and development, plants have developed mechanisms to maintain low levels of salt in the cytoplasm. One mechanism involves removal of Na+ from the cytoplasm by transport into the vacuole or out of the cell. This transport is catalyzed by Na+/H+ exchangers (antiporters), membrane proteins localized in the vacuolar (tonoplast) and plasma membrane, respectively [Figure 2 – model of plant cell]. Na+/H+-exchange activity is driven by the electrochemical gradient of protons (H+) generated by the H+-pumps in the plasma membrane (H+-ATPase) or the tonoplast (H+-ATPase and H+-pyrophosphatase). Our studies are focused on understanding the role and regulation of these transport proteins in the plant's response to salt stress.
Recently, much has been learned about signal transduction pathways that regulate plant ion homeostasis during salt stress (5). In a genetic screen designed to identify components of the cellular machinery that contribute to salt tolerance in Arabidopsis thaliana, three Salt-Overly-Sensitive genes (SOS1, SOS2, and SOS3) were found to function in a common pathway (6-9) [Figure 3 – SOS model]. Loss-of-function mutations in SOS1 render plants extremely Na+-sensitive, and overexpression of SOS1 in Arabidopsis improves salt tolerance (10). As was found for mutations in SOS1, mutations in SOS3 or SOS2 cause plant hypersensitivity to Na+ (8). SOS3 encodes an EF-hand type calcium-binding protein that may sense calcium changes elicited by salt stress (8). Calcium, together with SOS3, can activate SOS2, a serine/threonine protein kinase (9). SOS1 encodes a 127 kilodalton membrane protein with 12 putative membrane-spanning domains and a long hydrophilic tail at the C-terminal end of the protein (12). The predicted membrane-spanning domains in the SOS1 protein display significant similarity to domains of plasma membrane-localized Na+/H+ exchangers from animal, bacterial, and fungal cells. Salt stress up-regulation of SOS1 is partly under control of the SOS3/SOS2 regulatory pathway (12). We have recently provided the first molecular identification of a plasma membrane Na+/H+ exchanger in plants (13). Comparing Na+/H+ exchange activity in plasma membrane-enriched vesicles isolated from wild-type and sos mutant plants, we have shown that SOS1 is a Na+/H+ exchanger -- exchange activity was significantly reduced in plasma membrane-enriched vesicles isolated from sos1 plants relative to activity in vesicles isolated from wild-type plants (13) [Figure 4 – comparison of exchange activity WT vs sos1]. We have also shown that the transport activity of SOS1 is post-translationally activated by SOS2/SOS3 as mutations in SOS2 and SOS3 led to reductions in plasma membrane Na+/H+ exchange activity, and transport in these mutants could be restored by adding constitutively active recombinant (activated) SOS2 protein kinase in vitro (13) [Figure 5 – comparison of exchange activity WT vs sos2, sos3] [Figure 6 – exchange activity ± SOS2 protein].
For a complete picture of intracellular Na+ regulation during plant growth in salt, it will be necessary to understand how the individual proteins that transport Na+ function together. Na+/H+ exchangers that are responsible for vacuolar compartmentation of Na+ have been characterized from several plant specie s. Overexpression of tonoplast Na+/H+ exchangers in Arabidopsis (AtNHX) increased Na+ compartmentation in vacuoles and improved tolerance in transgenic plants (14-17). When compared with tonoplast Na+/H+ exchange activity in wild type, activity originating from AtNHX proteins was significantly higher, greatly reduced and unchanged in sos1, sos2 and sos3, respectively (18). Activated SOS2 protein added in vitro increased tonoplast Na+/H+ exchange activity in vesicles isolated from sos2 but did not have any effect of activity in vesicles isolated from wild type, sos1, or sos3. Our results demonstrate that (i) the tonoplast Na+/H+ exchanger in Arabidopsis is a target of the SOS regulatory pathway, (ii) there are branches to the SOS pathway, and (iii) there may be coordinate regulation of the exchangers in the tonoplast and plasma membrane.
To keep cytoplasmic levels of Na+ low, plant cells require the concerted activity of a number of transporters including the H+-pumps to provide the driving force for Na+ transport. Many of the transporters that would be required for the cellular response to excess Na+ have been identified in plants; however, little is know about their regulation. With the recent demonstration of SOS1 as a target of the SOS3-SOS2 pathway, we have the first insight into mechanisms underlying regulation of cellular Na+ homeostasis during salt stress. Current studies are focused on determining if the H+-pumps are also targets of the SOS pathway using in vitro, in vivo and in planta assays. As additional targets are identified, we will determine if there is coordinate regulation of pathway components during abiotic stress signaling.
Genetic and biochemical studies have demonstrated that SOS1 (a plasma membrane Na+/H+ exchanger) is critical for maintenance of cellular Na+ homeostasis and salt tolerance in Arabidopsis. Analysis of the deduced amino acid sequence of the SOS1 protein has shown that it contains a long C-terminal tail that is likely to be localized in the cytoplasm. Recently, a regulatory function for the C-terminus of a SOS1-like protein (ApNhaP) from the cyanobacterium Aphanothece halophytica has been demonstrated (19). ApNhaP is an exchanger with novel ion specificity in that it displays both Na+ and Ca2+-coupled H+ transport (exchange). When the long C-terminus of ApNhaP was replaced with the C-terminus from a related transporter from Synechocystis with different ion specificity, the ion specificity of the Aphanothece protein was changed. This result indicates that the C-terminus of ApNhaP contains structural elements that are critical for ion-specific binding. We are determining the function of the C-terminus in SOS1 by asking if it plays a role in the regulation of SOS1 transport activity. To answer this question, we are determining the ion-specificity of SOS1 and the effects of altered forms of the SOS1 C-terminus on ion transport and salt tolerance in vivo and in planta.
Identifying the genes, the biochemical mechanisms and the signaling pathways that mediate salt tolerance in a halophyte
Identifying salt tolerance elements has been the subject of research for decades; a typical approach has included a comparison of growth and physiological parameters in glycophytes grown in the absence and presence of salt, looking for responses to added salinity. The difficulty with such an approach is that it is not possible to distinguish those responses that are truly adaptive from those that are reflections of metabolic lesions. In many halophytes, the optimum salinity for growth is shifted to levels of salt at which most plants and all crop plants experience severe reductions in growth and yield [Figure 7 – Greenway and Munns] (20). As a result, halophytes provide the opportunity to compare growth at low and high levels of salinity to distinguish responses that are truly adaptive from those that are the result of lesions or other types of damage. Research with halophytic species has provided glimpses of these adaptive components, but has been limited by the lack of molecular genetics in any of the species studied. With the advent of molecular genetics in Arabidopsis, functional studies have identified genetic elements and pathways that alter stress sensitivity in this glycophyte. Using information from studies in Arabidopsis, we can now determine if these components (salt and osmotic tolerance determinants) and mechanisms (the associated regulatory pathways) are part of the adaptations used by Thellungiella halophila (salt cress), a naturally salt tolerant, genetically tractable relative of Arabidopsis (21) [Figure 8 – salt cress ± salt]. We are eliminating genes from salt cress that have been identified as important for stress tolerance in Arabidopsis. These studies will allow us to determine the extent to which these elements, identified in glycophytic model species, play a role in the life of a halophyte and will also allow us to generate transgenic models for comparative studies of salinity stress responses. In addition, we are determining the capacity of gene products and promoters from halophytic salt tolerance genes to mediate functional sufficiency for salt adaptation in a glycophyte. The Arabidopsis genome includes most of the genes that have, been associated with tolerance in other species, yet Arabidopsis is not tolerant to salinity or drought. One way to interpret this is that, while Arabidopsis possesses the necessary genes for stress tolerance, the regulatory mechanisms (controlling how strongly, when, and where the tolerance genes are engaged) are absent, incomplete, or inefficient. These studies will enable us to functionally identify the genes, the biochemical mechanisms and the signaling pathways that mediate tolerance in a halophyte.
Mechanisms controlling stimulus-induced changes in intracellular calcium
Calcium (Ca2+) has emerged as a critical component of many pathways in plants, underlying growth and development by linking perception of physiological and environmental cues to cellular responses. Because cellular Ca2+ levels are tightly regulated, small changes in cytosolic Ca2+ levels provide information for the modification of enzyme activity and gene expression needed for the subsequent responses. Stimulus-induced changes in intracellular Ca2+ are generated by transport proteins that allow the downhill flow of Ca2+ from a compartment in which the ion is present at high electrochemical potential (either outside the cell, or in the lumen of the vacuole or endoplasmic reticulum) into the cytoplasm in which Ca2+ is at lower potential. Numerous studies have implicated phosphoinositide-induced intracellular Ca2+ release (PICR) as part of the mechanism leading to stimulus-induced increases in intracellular Ca2+ levels in plants. Many of the components of the PICR pathway, including the intracellular messenger inositol 1,4,5-trisphosphate (InsP3), have been identified in plants as a result of genetic, biochemical and pharmacological studies. What is missing is the molecular identification of the InsP3 receptor (InsP3R), a Ca2+ channel that is responsible for these stimulus-induced increases in intracellular Ca2+.
In animal cells, the InsP3R is regulated by InsP3 and Ca2+ and by a family of Ca2+ sensor proteins (CaBPs, 22). The CaBPs bind with high affinity to the InsP3-binding region of the InsP3R and function as agonists of the InsP3R in the presence of Ca2+ but in the absence of InsP3. These animal CaBPs share significant homology with the SOS3 and the SOS3-like (SCaBP1-9) family of calcium binding proteins in Arabidopsis.
The recent identification of calcium binding proteins as novel protein ligands for the InsP3R in animal cells and the presence of plant calcium binding proteins with similar features and properties, provide an important new strategy to identify the receptor -- a component of the mechanism underlying Ca2+ regulation in plants. We are taking biochemical, molecular biological and genetic approaches to determine if the Arabidopsis CaBPs release Ca2+ from intracellular stores, if they interact with the InsP3R and to determine potential sites of CaBP/InsP3 interaction in the plant and in the cell. Identification of this receptor will be critical for understanding the specificity of Ca2+ signaling in plants and ultimately for designing strategies to modify the responses of plants to physiological and environmental cues.
References
1. O'Leary, J.W. 1995. Adaptive Components of salt tolerance. In: Handbook of plant and crop physiology. Mohammad Pessarakli, ed. Marcel Dekker, Inc, NY, pp. 577-585. 2. Rhoades, J.D. and Loveday, J. 1990. Salinity in irrigated agriculture. In: American Society of Civil Engineers, Irrigation of Agricultural Crops (Steward, B.A. and Nielsen, D.R., eds), Am. Soc. Agronomists, Monograph 30, 1089-1142.
3. Evans, L.T. 1998. Feeding the Ten Billion. Cambridge University Press, 247 p.
4. Penning de Vries, F.W.T. 2001. Food security? We are loosing ground fast! In: Nösberger J et al., eds. Crop Science: Progress and prospects, CABI Internatl Publ., pp. 1-14.
5. Zhu, J.-K. 2002. Salt and drought stress signal transduction in plants. Annu. Rev. Plant Biol. 53, 247-273.
6. Wu, S.-J., Lei, D. and Zhu, J.-K. 1996. SOS1, a genetic locus essential for salt tolerance and potassium acquisition. Plant Cell 8, 617-627.
7. Liu, J. and Zhu, J.-K. 1997. Proline accumulation and salt-stress-induced gene expression in a salt-hypersensitive mutant of Arabidopsis. Plant Physiol. 114, 591-596.
8. Zhu, J.-K., Liu, J. and Xiong, L. 1998. Genetic analysis of salt tolerance in Arabidopsis: evidence for a critical role of potassium nutrition, Plant Cell 10, 1181-1191.
9. Zhu, J.-K. 2000. Genetic analysis of plant salt tolerance using Arabidopsis. Plant Physiol. 124, 1-8.
10. Shi, H., Lee, B.-H., Wu, S.-J. and Zhu, J.-K. 2003. Over expression of a plasma membrane Na+/H+ antiporter improves salt tolerance in Arabidopsis. Nature Biotech. In press.
11. Halfter, U., Ishitani, M. and Zhu, J.-K. 2000. The Arabidopsis SOS2 protein kinase physically interacts with and is activated by the calcium-binding protein SOS3. Proc. Natl. Acad. Sci. USA. 97, 3730-3734.
12. Shi, H., Ishitani, M., Wu, S.-J., Kim, C.-S. and Zhu, J.-K. 2000. A sodium-proton antiporter functions at the xylem/symplast boundary to control plant salt tolerance. Proc. Natl. Acad. Sci. USA. 97, 6896-6901.
13. Qiu, Q.-S., Guo, Y., Dietrich, M.A., Schumaker, K.S. and Zhu, J.-K. 2002. Regulation of SOS1, a plasma membrane Na+/H+ exchanger in Arabidopsis thaliana, by SOS2 and SOS3. Proc. Natl. Acad. Sci. USA 99:8436-8441.
14. Apse, M.P., Aharon, G.S., Snedden, W.A. and Blumwald, E. 1999. Salt tolerance conferred by overexpression of a vacuolar Na+/H+-antiport in Arabidopsis. Science 285, 1256-1258.
15. Blumwald, E. 2000. Sodium transport and salt tolerance in plants. Curr. Opin. Cell Biol. 12, 431-434.
16. Zhang, H.-X. and Blumwald, E. 2001. Transgenic salt tolerant tomato plants accumulate salt in the foliage but not in the fruits. Nature Biotech. 19, 765-768.
17. Zhang, H.-X., Hodson, J., Williams, J.P. and Blumwald, E. 2001. Engineering salt-tolerant Brassica Plants: Characterization of yield and seed oil quality in transgenic plants with increased vacuolar sodium accumulation. Proc. Natl. Acad. Sci. USA 98, 12832-12836.
18. Qui, Q.-S., Guo, Y., Quintero, F.J., Pardo, J.M., Schumaker, K.S. and Zhu, J.-K. 2004. Regulation of vacuolar Na+/H+ exchange in Arabidopsis thaliana by the Salt-Overly-Sensitive (SOS) pathway. J. Biol. Chem. 279, 207-215.
19. Waditee, R., Hibino, T., Tanaka, Y., Tatsunosuke, N., Incharoensakdi, A. and Takabe, T. 2001. Halotolerant cyanobacterium Aphanothece halophytica contains a Na+/H+ antiporter, homologous to eukaryotic ones, with novel ion specificity affected by C-terminal tail. J. Biol. Chem. 276, 36931-36938.
20. Greenway, H. and Munns, R. 1980. Mechanisms of salt tolerance in nonhalophytes. Ann. Rev. Plant Physiol. 31, 149-190.
21. Zhu, J.-K. 2001. Plant salt tolerance. Trends Plant Sci. 6, 66-71.
22. Yang, J., McBride, S., Mak, D.-O.D., Vardi, N., Palczewski, K., Haeseleer, F. and Foskett, J.K. 2002. Identification of a family of calcium sensors as protein ligands of inositol trisphosphate receptor Ca2+ release channels. Proc. Natl. Acad. Sci. USA 99, 7711-7716.
home | who we are | research | publications | tools | contact us
|